Traffic-Aware Resource Allocation and Performance Analysis
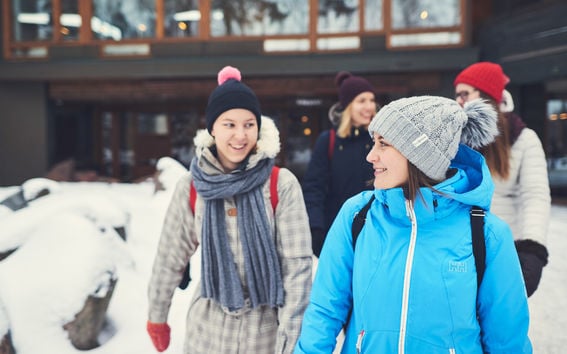
Research challenges
Intercell-coordination
The dense deployment cells creates severe intercell-interference problems, especially for cell edge users. Also, if 5G networks will utilize much higher frequencies than LTE systems, this may affect the way that the impact of interference is modeled. We develop novel policies for controlling the resource allocation of the base stations at a fast time scale depending on the traffic load in order to optimize the performance. For elastic traffic, the modeling results in a system coupled PS queues with state dependent service rates.
Load balancing between femtos cells and the macrocell
In a typical scenario, one can consider a macrocell with multiple small cells (femtos, picos, …) inside the macrocell coverage area. A given user in the coverage area of some small cell has the option to be served locally by the small cell or by the macrocell. From the point of view of elastic traffic each cell can be modelled as a processor sharing queue and the problem becomes a so-called generalized dispatching problem where the macrocell performs as an overflow channel.
Performance-energy trade-off in 5G networks
Energy efficiency is a growing concern for the whole ICT industry. Fundamentally, performance and lower energy usage represent a tradeoff and this aspect can always be included in the studies. However, the context always sets its own requirements for the modeling. For example, in the macrocell/small cell scenario the macrocell always needs to be on in order to have coverage, but the small cells can potentially be switched off to save energy.
Impact of massive M2M data
The vision of IoT brings a new type of traffic to the system known as machine-to-machine (M2M or MTC) communication. In this scenario, sensors (or small devices in general) send periodically small messages to some remote facility but within the cell area there may be thousands of them. In such cases, the signaling resources of the LTE (or future 5G) system may become a bottleneck preventing stations from accessing the network. The signaling channel of the system behaves more like a multichannel slotted Aloha system. This needs to be taken into account, e.g., in the load balancing scenario.
Green data centers
According to the C-RAN architecture, data centers will also be an important building block in the architecture of the 5G cellular network architecture. A network of (possibly heterogeneous) servers with ultra high-speed connections comprises the core of a data center. Dispatching an arriving job to a server is a fundamental task in such context. In order to save energy, it may be reasonable to switch off idle servers. However, switching them back on takes typically a long time (so called startup delay), thus, deteriorating performance, which may even collapse. Our purpose here is to model and analyze this kind of performance-energy trade-off in data centers, and develop efficient policies for dispatching jobs and switching servers on and off, as well as for optimal placement of virtual machines.
Example of recent research results
In the presence of a large number of M2M devices, the actual resources allocated for the signaling channel in LTE may become a bottle neck. This has been analyzed in our recent paper [1]. Essentially, the access consists of a four-way handshake with the network, see left figure below. The system can be modeled a multichannel slotted Aloha channel at the first step (Message 1) but with some additional limiting factors accounting for the impact of later steps (Messages 2, 3 and 4). In the paper, we prove for example the stability limit of the system that characterizes the maximum throughput (number of successful access attempts per time unit). This result is illustrated in the right figure below. It shows the various throughput curves as a function of the offered load “a” for a certain selection of the system parameters. The purple curve represents the throughput of successful Message2 transmission per time unit, while the green curve depicts the mean service rate available for the Message2’s after Message4’s have been taken care of. The intersection of these gives the maximum rate of successful access attempts, i.e., the overall maximum throughput per time unit of the system.
[1] P. Osti, P. Lassila, S. Aalto, A. Larmo and T. Tirronen, “Analysis of PDCCH performance for M2M traffic in LTE”, IEEE Transactions on Vehicular Technology, vol. 63, no. 9, p. 4357-4371, 2014.
- Published:
- Updated: